SEM Lab, Inc. supports printed wiring board failure analysis and construction analysis. This presentation documents some of the most common failure causes seen in this laboratory. These include Hi-Pot failures, lamination failures, inner layer separation, corrosion failures, PTH Cu-plating failures, and conductive anodic filament failures. These failures can be mitigated to a significant degree by utilizing construction analysis to verify PWB quality. Construction analysis allows assessment of conductor layer thickness, dielectric layer thickness, PTH copper thickness, layer-to-layer registration, standard via & micro-via quality, PTH fill quality, and drilled-hole quality to name a few critical feature attributes. Download >>> Failure Analysis of PWBs
These are some representative examples of corrosion failure of electronic components and assemblies characterized in this laboratory.
Example 1: This is a BSE SEM image of a contact tine from a telecommunications jack. The tine is gold-plated over a nickel-underplate over copper alloy. The primary corrosion product was copper oxide that indicated corrosion of the tine base metal. Corrosive agents were found on the surface including sodium and potassium hydroxides, which in the presence of moisture can result in galvanic attack of the base metal through minute defects (pores and seams) in the plating resulting in the type of damage observed here.
Example 2: This is an electro-mechanical relay that failed to function due to internal corrosion where the corrosion product (rust) prevented the armature from fully actuating. Bromine was found in the corrosion product, which likely accelerated the corrosion damage.
Example 3: This is an example of severely corroded PTH-vias as viewed from the PWB surface and in cross-section. The corrosion appears to have been caused by residual chloride from a highly activated soldering flux. The un-filled vias easily trap process chemicals including solder flux. Another example of a corroded PTH-via is shown here.
Example 4: This is an aluminum bond pad on a failed plastic-molded op amp that was chemically decapsulated. The aluminum bond pad had been nearly completely etched away due to internal corrosion, which resulted in failures due to increased electrical resistance. Some aluminum remained in the corners of the pad (arrows). CSAM imaging showed internal separation of the molding compound from the die surface and lead frame. In addition, fractures were found in the plastic molding compound suggesting that the parts were damaged by “popcorning” during solder reflow.
Corrosion related electronics failures are common in this laboratory’s experience.
These are some representative solder joint failure modes found in this laboratory that illustrate
* mechanical overload at high strain rate,
* thermal fatigue accelerated by gold embrittlement,
* creep rupture failure
Example 1: This is an SMT thick film resistor solder joint. The solder joint failed in a brittle fracture mode at the interface between the solder and the nickel barrier plating (i.e. brittle interfacial fracture). The analysis results suggested that the resistor failure was most likely caused by mechanical stress (likely in bending) at a high strain rate.
Example 2: This example shows a thermal fatigue fracture. The solder alloy is SN63, the package is a J-lead PMIC, and it is soldered to an alumina substrate. The thermal fatigue fracture showed classic characteristics such as grain boundary separation and propagation through the bulk solder joint. In this case, the failure was accelerated by gold embrittlement of the solder joint (bulk solder joint contained ~ 3 wt% of gold). Fracture is driven by cyclic creep-fatigue damage due to elastic displacement of the leads being converted to time-dependent plastic (creep) strain in the solder joint during thermal cycling. The cyclic strain is due to CTE mismatch between the PMIC and the ceramic substrate.
Example 3: This example shows a creep rupture failure of an SMT connector solder joint. The lead that failed was under stress as-soldered. The vertical displacement of the lead after the solder joint fractured is the key feature that suggests this was a creep rupture failure. The elastic strain of the lead is converted to creep strain in the solder joint until it either ruptures or the stress is relieved.
It is important to identify the failure mode accurately in order to formulate appropriate corrective actions.
This is a BSE SEM image of a glass rectifier diode that was chemically decapsulated to remove the glass case.
This is the diode die, which showed two separate breakdown sites that likely occurred simultaneously.
The die fractures are due to thermal shock due to the thermal effect rapid quenching by the surrounding die material immediately after the event.
This type of breakdown is likely due to a fast voltage transient.
BGA warpage can cause a condition called “pad cratering”.
This condition occurs when the assembly cools down below the solidus temperature after reflow soldering and the solder joint is put under tensile loading that exceeds the cohesive strength of the PWB laminate.
The condition often leads to open circuit failures that affect assembly yields.
BGA solder joint microsections are useful for evaluating microstructure, interfacial intermetallic layer thickness, voids, and other conditions. The example below shows a nominal BGA solder joint on 0.8 mm pitch.
In addition, the geometry of the solder joint can provide useful data such as deviation from the nominal condition and the magnitude of residual warpage.
For the above example, i.e. nominal BGA solder joint geometry, the parameters of the solder joint geometry are …
Nominal SJ Height (mm) = 0.25
PWB Pad Dia. (mm) = 0.50
BGA Pad Dia. (mm) = 0.40
h2 (mm) = 0.10
h3 (mm) = 0.15
r1 (mm) = 0.30
Solder Vol. (mm^3) = 0.0661
In summary, there is much to be learned from BGA solder joint microsections.

MICROSECTIONS
The terms microsections and cross-sections and metallography all refer to essentially the same process, which has been in use for a very long time. This metallurgist was first involved in preparation of metallurgical sections in 1979 while working as a Project Engineer for Northwest Technical Industries, which is now PA&E’s Bonded Metals Division. Metallographic cross-sections such as the one shown in Fig. A were prepared using standard metallographic practices to document the “wave pattern” and other features of the explosively bonded material.
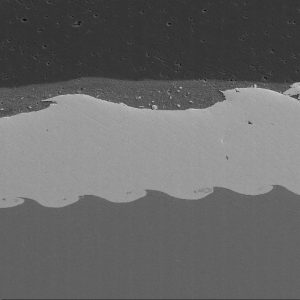
The same standard metallographic practices are used to microsection electronic components, printed wiring boards, solder joints, etc., which are typically combinations of metallic, ceramic, and polymeric materials. Some examples are shown below.
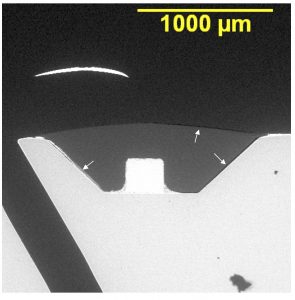
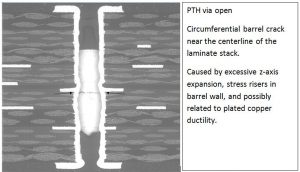
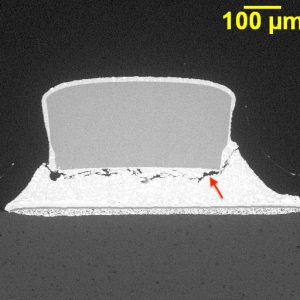
Preparation of microsections is more difficult for combinations of soft materials (metal & polymers) and hard materials (ceramic & semiconductors) because the soft materials polish at a faster rate than the harder materials. Nevertheless, it is worth the effort because root cause failure analysis often requires examination of microscopic material details such as microstructure, grain size, porosity, deformation, etc. that would be difficult or impossible to examine using other techniques. Measurements of solder joint height in microsections can be used to calculate the degree of thermal-mechanical warpage in a BGA assembly as illustrated below.

Multilayer chip capacitors (MLCCs) can fail due to a manufacturing defect known as Knit Line Fracture, which is a lamination defect causing separation on internal layers.
Check out SEM Lab, Inc. to learn more.
In the previous two decades, black-pad-syndrome was a leading cause of intermittent BGA electrical failure. In 2018, this seems to have been replaced by head-in-pillow defect. Both of these conditions generally require microsection analysis for a conclusive diagnosis as non-destructive techniques mostly fail to identify a problem. Here are some examples of head-in-pillow defect analyzed at SEM Lab, Inc.
Head-in-pillow defect at ball-A3
Head-in-pillow defect at ball-B5
This condition is primarily caused by thermal-mechanical warpage of the BGA during the solder reflow process. Some corrective action recommendations can be found in [1].
[1] SMT Troubleshooting Guide, “BGA Head-on-Pillow”, www.alpha.cooksonelectronics.com, Issued 10/08, p. 17.
Recent Posts
- PCBA bending
- Failure Analysis vs Testing
- Electronic Connectors
- LED Failures
- Vias in Printed Circuit Boards
- Flux Cleaning is Essential
- Premature Failure of WLEDs
- ENIG Analysis
- Diode bridge
- BGA Solder Joint Simulation
- QFN Failures
- PWB Quality Problems
- Al Bond Pad with Ti-W Barrier
- Thin Film Thickness Measurements
- EOS fused bond wire
- EOS Damaged Op Amp
- BGA Assembly Verification
- Contamination on MLCC Termination
- MLCC Knit Line Related Failures
- CMOS Flip-Flop IC
- Failure Analysis of PWBs
- Corrosion Failures
- Solder Joint Failure Modes
- Glass Rectifier Diode
- BGA Pad Cratering
- BGA Solder Joint Microsection
- BGA Solder Joint Height
- Microsections
- MLCC knit line failure
- Head-in-pillow defect
- IR LED Open Circuit
- Dual Op Amp
- MELF Resistor
- SMT Transformer
- LED Bond Wire Break
- Chip Components
- Microcontroller failure
- QFN Solder Joint Evaluation
- Connector Contamination
- Thermal Stress or EOS
- PWB Short
- Creep Rupture Failure
- Mechanical Overstress of Resistor Solder Joints
- BGA warpage
- VD on PCB
- Pb-dendrites
- Corrosion of Flex Assembly
- Optical vs SEM imaging
- FTIR of Unknown Plastic
- Counterfeit AE Capacitors
- Nichrome Resistors
- Yellowed resistors
- ENIG Discoloration
- Laminate Damage
- Wear Out of Aluminum Electrolytic Capacitor
- Suspected tin whisker
- Solder Joint Fatigue
- Failure of IR LED
- Residue on PCBA
- MLCC Bending Fracture
- FPGA ECM short
- Tin Whiskers
- LED Lamp Flickers
- Gold Embrittlement
- MLCC manufacturing flaw
- Diode Short
- ESD Damage in Zener Diode
- Severe corrosion on PCBA
- PCBA Corrosion
- Shorted FET
- Dendritic Growth – Shorts on PCBA
- ECM of PWB Battery Contacts
- Bad Board Design
- Solder Joint Failure
- Tombstoning of SMD Inductor
- Silver Sulfide Crystals
- IC Bond Pad Corrosion
- Crystal Frequency Shift
- Damage in BGA Device
- Manufacturing flaws in ceramic capacitor
- Red Phosphorus in IC Molding Compound
- ENIG corrosion problem
- FET failure
- Diode failure
- Ta-cap breakdown
- MLCC bending fracture
- Internal PWB Short
- Microsection of gold-plated contact
- Trace fractured on Rigid Flex
- EOS failure of Power MOSFET
- Black Pad Syndrome
- Power Transistor
- Blue LED
- Tin Whiskers
- Bad diodes
- EDS shows Teflon Contamination
- Tin whisker
- Residue under BGA
- Fuses on Video RAM
- Solid-State Relay
- Knit Line Fracture and Porosity
- ESD damaged diode
- Silver Sulfide Growth
- MLCC short
- Fused bond wire
- Skip plating
- Resin Starvation
- Quad NOR ICs
- LED Failure
- Electromigration short
- Stranded wire failure
- Corroded PTH-via
- Tin-lead whisker
- SAC solder joint
- SMD diode short
- Excess solder gull-lead joint
- Decapsulated diode
- BGA die crack
- EOS on GaAlP LED
- BGA warpage
- EOS Dual Op Amp
- Double-Dumet diode
- Corroded inductor windings
- EOS damage on VDD
- EOS fused bond wire
- Marking on transistor die
- Welded relay contact
- Inner Layer Separation
- Bond cratering microsection
- MLCC dielectric porosity
- Diode EOS
- MLCC flaws
- Parylene coating
- Diode EOS
- BGA gas voids
- Distorted BGA
- PLCC bond fail
- Conductive residue
- PTH barrel crack
- Missing BGA ball
- LED die fracture
- Fractured solder joint
- Electromigration short
- MLCC firing cracks
- Ta-cap failure
- Over crimped
- Suspect wedge bond
- BGA ball fail
- Bad HASL
- LED bond failure
- LED failure
- Resistor network solder joint fracture
- PTH via barrel crack
- EOS failure
- ENIG problem
- Black pad syndrome
- Poorly drilled hole
- IC voltage transient
- Wire bond
- Bond cratering
- Chip resistor fracture
- Via corrosion
- MLCC knit line failure
- SOT23 diode
- Chip resistor corrosion
- BGA Solder Mask Defined Pads
- PTH Pad Lift
- LED microsection
- MLCC flex fracture